Neutron stars
- Jay Sardesai
- Dec 3, 2021
- 4 min read
Neutron stars are created after the collapse of massive stars, with a mass greater than the Chandrasekhar mass (at least 8 times more massive than the Sun, but varying according to the star). This collapse is known as a supernova, and has a luminosity of up to 5 trillion times that of the Sun. The iron-rich core left behind is incredibly dense, roughly similar to the density of an atomic nucleus, and has an incredibly strong magnetic field.
The neutron star consists only of the core of the star, and not the outer layers; while most stars which become neutron stars have a mass of between 10 and 29 solar masses, the neutron stars themselves have only a mass of roughly 1.4 solar masses on average. In addition, due to the extreme increase in density, the neutron star's radius decreases to ~20km on average. As a result of the conservation of angular momentum, because the outer layer of the star is so much closer to the centre, it must move much faster, making neutron stars (especially newly formed neutron stars) rotate very quickly. Most neutron stars slow down over time(spin down); this is presumed to be roughly equal to the luminosity of magnetic dipole radiation of the given star.
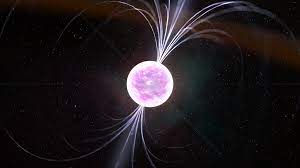
There are, however, some examples of spin up neutron stars, where their rotation has increased over time. This is most common when neutron stars take matter from orbiting stars, drastically increasing their rate of rotation. This is the known method by which millisecond pulsars (pulsars with a rotational period of <40 milliseconds) are created; due to the existence of millisecond pulsars with very high magnetic fields, a second process must exist, but it is currently unknown.
The atmosphere of a neutron star is only a few micrometres thick due to the extremely strong gravity of neutron stars. The outer crust consists of atomic nuclei forced into a regular lattice-like arrangement with electrons free to flow between them. The structure of the inner rust is hypothesised to resemble "nuclear pasta". In the outermost layer, the pressure is so great that nuclei are forced together into collections of nuclei, resembling much larger atoms, in what is called the "gnocchi" phase. As the pressure increases further inside the star, the nucleons are forced into much larger groups, resembling spaghetti, and as the pressure increases even more, these join to form "lasagna" like sheets. As each stage, the percentage of nucleons which are neutrons increases. Eventually, in the outer core, the electrostatic repulsion between protons and electrons is overcome by the extreme pressure, and the core is composed entirely of neutrons. Little is known about this state of matter, and there are yet more theories about the hypothesised inner core. Suggestions include an exotic form of quark-gluon plasma as the strong force is no longer able to hold nucleons together (this occurs at the Hagedorn temperature of 1.7*10^12 Kelvin, and has been observed in particle collisions at the LHC), however, currently there is no way of proving that this exists at the centre of neutron stars
Pulsars are a type of neutron star where the magnetic fields funnel jets of charged particles and radiation out along the magnetic poles. As the poles of the star are usually not aligned with its axis of rotation, the radiation beam can only be seen once every rotation by Earth. This allows astronomers on Earth to find the speed at which the pulsars rotate. In addition, the beams allow astronomers to identify the stars as neutron stars, which is why most (2000/3000) of observed neutron stars are pulsars, as, without the radiation beam, they are very hard to detect.
Magnetars are a type of neutron star with a very large magnetic field, of roughly 1-100 billion Tesla ( for comparison, the Earth has a magnetic field strength on the surface of 0.025 to 0.065 Teslas). To demonstrate the ridiculous size of the magnetic field, the energy density of an area with a magnetic field strength of 10 billion Tesla would be roughly 4.0*10^25 J/m^3. Using E = mc^2, we can see that this would give it a mass density of 4.4*10^8kg/m^3, an absurdly high density. Although magnetars are predicted to make up at least 10% of all neutron stars, only about 1% (29/3000) of known neutron stars are magnetars, and only 6 are both magnetars and pulsars. Magnetars are considered to be "soft gamma repeaters"; they emit bursts of gamma rays and x-rays at irregular intervals. SGR 1806-20 is an example of a soft gamma repeated, first observed in 1979
Occasionally, when both stars in a binary system are massive enough to become neutron stars, a neutron star binary system is formed. Over time, these stars drift closer and closer together, until they collide in a kilonova. This kilonova has a luminosity roughly 1000 times greater than a regular nova (where a star suddenly "appears" in the sky and then fades, usually due to a white dwarf in a binary system stripping gas from its partner) and 10 to 100 times greater than a supernova. Kilonovas are at least partially responsible for the production of almost all elements of number 41(niobium) and higher, and are exclusively responsible for the production of elements between 83 (bismuth) and 94(plutonium) through the rapid neutron-capture process.
The rapid neutron-capture process involves the rapid addition of neutrons to a nucleus (most commonly iron) before the nucleus can decay to a more stable atom. This process only occurs where there is a very high abundance of neutrons, such as in the collision of neutron stars. This process has also been observed in the "Ivy Mike" thermonuclear test by the US, where einsteinium(99) and fermium(100) were observed in the fallout. As a result, without neutron stars, we wouldn't have many heavy elements, such as uranium, and others, like gold and platinum, would be much rarer. Therefore, even though we do not live near a neutron star, they have still affected us greatly.
Sources:
Comments