Introduction to the Standard Model
- Jay Sardesai
- Oct 22, 2021
- 5 min read
The Standard Model is a theory used to describe the interactions of three of the fundamental forces(strong, weak, and electromagnetic) with the 17 fundamental particles. These particles are divided into two groups: fermions and bosons.
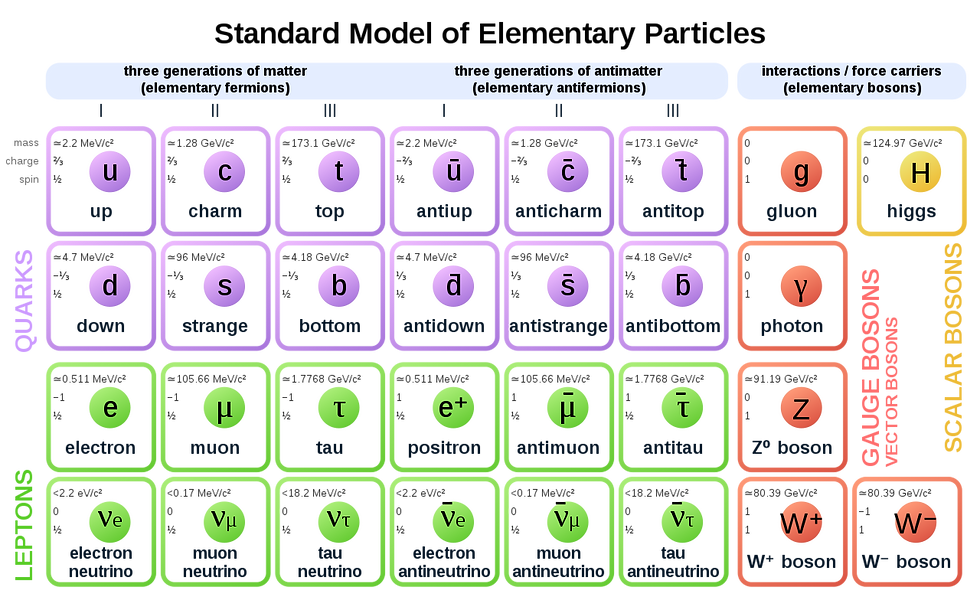
Fermions are particles which follow Fermi-Dirac statistics. They have half-integer spin (e.g. 1/2, 3/2) and so cannot occupy the same quantum state in the same space as an identical fermions. This is why only two electrons can occupy each subshell. Fermions comprise quarks, leptons, and particles composed of an odd number of fermions (particles composed of other particles are called composite particles) such as protons and neutrons. Particles composed of an odd number of composite fermions are themselves fermions, such as helium-3.
There are six different 'flavours' of quarks: up, down, charm, strange, top and bottom. These are further subdivided into three generations, each containing two quarks, where properties remain mostly the same but mass increases. The three generations are: up + down, charm + strange, top + bottom. In each generation, one quark(up, charm, top) has a charge of +2/3(relative to the charge of an electron), and the other(down, strange, bottom) has a charge of -1/3. There are also six leptons, divided again into three generations of increasing mass. Each generation consists of a charged lepton (electron, muon, tau) and a chargeless lepton, called a neutrino(electron neutrino, muon neutrino, tau neutrino). The neutrinos are sometimes given the names nu_L, nu_M, and nu_H (lightest neutrino, middle neutrino, and heaviest neutrino). Each lepton has a spin of 1/2, and charged leptons have a spin of -1. Each fermion also has a corresponding anti-particle (anti-up, anti-down etc.) with the same mass and spin but opposite charge. In addition to electromagnetic charge, there is also a similar weak isospin, for the weak force, with two components T and T3, and colour charge, for the strong force, with three components red, green and blue.
Bosons are particles which follow Bose-Einstein statistics and have integer spin (1, 2). Bosons are split into gauge bosons (Z, W, photon and gluon), and scalar boson (Higgs). Bosons also include composite particles composed of an odd number of fermions, such as mesons (composed of a quark-antiquark pair). Each vector boson is a carrier of a certain force: the W and Z bosons are responsible for the weak interaction, the photon for the electromagnetic interaction, and the gluon for the strong interaction. While a force carrier for gravity, the graviton, has been suggested, its existence has not been proven.
The weak interaction is divided into two different interactions: charged-current interaction and neutral-current interaction. Charged-current interaction involves the emission of a W+/W- boson, leading to a change in charge of the original particle. The W boson then decays rapidly into two fermions, either an (anti)electron and (anti)neutrino, or quark-antiquark pair, such that charge is conserved (e.g. a W- boson can decay into an electron and antineutrino (overall charge stays -1), but not a positron(antielectron) and neutrino, as the overall charge would change). The most relevant example of charged-current interaction is beta decay, of which there are two forms: β- and β+. To understand beta decay, we first need to understand the composition of protons and neutrons. Protons and neutrons are not elementary particles, but are composite particles composed of three quarks (a type of baryon) bound together by the strong force. Protons contain two up quarks and one down quark, while neutrons contain one up quark and two down quarks, giving them their respective charges of +1 and 0. In β- decay, a down quark in a neutron emits a W- boson. As a W- boson has a charge of -1, and the down quark had a charge of -1/3, the new quark must have a charge of +2/3 to conserve charge, and so the down quark is converted into an up quark. This means that the baryon now has 2 up quarks and 1 down quark, and so is a proton. The W- boson then decays into an electron and antielectron neutrino. β+ decay involves the emission of a W+ boson by an up quark, leading to the conversion of a proton to a neutron, by the same process as detailed above. Charged-current interaction is also responsible for muon and tau decay, in which the particle emits a W- boson, becoming its respective neutrino. The W- boson then decays into an electron and antielectron neutrino. Neutral-current interaction involves the emission of a chargeless Z boson (which does not change the mass, colour, or charge of the particle) during the interaction between two leptons.
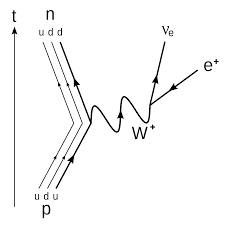
The photon is the force carrier for electromagnetic interactions. In an electromagnetic interaction, such as that between two electrons, a virtual photon is exchanged between the particles, leading to a change in direction of both electrons by a transfer of momentum. Photons are only able to interact with charged particles, such as electrons, and as such photons cannot interact with other photons. The gluon is the force carrier of the strong force, and possesses a colour charge itself, and as such gluons are able of interacting with other gluons. The idea of 'colour' was invented such that three otherwise identical fermions, subject to the Pauli Exclusion principle, would be able of existing in the same space within a baryon, such as an Ω- baryon (composed of three strange quarks). As the three quarks must be different in some way, they must have another property, with three components, dubbed 'colour'. 'Anti-colour' also exists, such as 'anti-red', just as electric charge can be both positive and negative. The strong force is mediated by the exchange of gluons between quarks. For example, a green quark can emit a green anti-red gluon, becoming red. The gluon is then absorbed by a red quark, which changes colour to green. This leads to a very strong attractive force between the quarks. The strong interaction also leads to some interesting effects, such as colour containment, where the energy required to separate a quark from a hadron is so great that a quark-antiquark pair is created, meaning that the removed quark is replaced, and a meson is created. As a result of this effect, it is impossible to isolate quarks from hadrons unless the temperature is greater than 1.7*10^12 K, which would destabilise the hadrons, separating the quarks.
The Higgs boson is the only scalar boson (yet) in the Standard Model. It was created to explain why the W and Z bosons have mass, by means of interacting with the Higgs field. According to the Brout-Englert-Higgs mechanism, mass is a result of interaction with the Higgs field, with a particle that interacts with the Higgs field more having a greater mass than one which interacts less. Under this theory, photons have zero mass as they do not interact with the Higgs field. An unknown particle of similar mass to the Higgs boson was observed at the LHC, meaning that the Higgs boson has now been widely accepted as part of the Standard Model. Gravitons are a hypothetical particle with the purpose of adding gravity to the Standard Model. Unfortunately, unlike the Higgs boson, their existence has not been proven yet, and there are significant mathematical difficulties with unifying gravity with the other three fundamental forces.
Sources:
Comments