Antimatter
- Jay Sardesai
- Sep 10, 2021
- 4 min read
Antimatter is made up of antiparticles. These antiparticles are almost identical to regular particles, except for one key difference: almost all of their quantum values are the opposite of regular matter (opposite electric charge, opposite colour charge, opposite spin and so on). Antimatter has been synthesised in very small amounts in particle accelerators, and is regularly used in Positron Emission Tomography. However, the cost of producing antimatter is currently so prohibitively high that building an antimatter bomb is not a sensible use of resources.
In medicine, Positron Emission Tomography (PET) uses unstable nuclei with a much higher number of protons than neutrons as a tracer. These nuclei then decay by positive beta decay, where a proton emits a W+ boson, becoming a neutron. The W+ boson then decays into a positron and electron neutrino. When released in the body, the positron almost immediately annihilates, releasing gamma rays. These gamma rays are then detected by a machine, allowing it to find where the tracer ended up in the body. Glucose substitutes are frequently used, as they concentrate in specific areas of the body with a very high metabolism, such as the brain and tumours, allowing the location of tumours to be found.
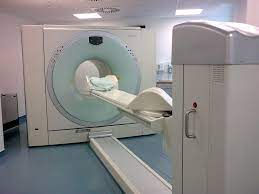
At CERN, the Antiproton Decelerator is used to slow down high-energy antiprotons created by collisions between a proton beam from the Proton Synchrotron. The antiprotons must be deflected and contained using magnetic fields: if they hit the sides of the tunnel, they would annihilate, releasing a large amount of energy. Multiple collisions of this form would cause significant damage to the magnets over time. The antiprotons are slowed down by magnetic fields until they reach approximately 1/10th of the speed of light.
CERN is also in the process of building a new deceleration ring, Extra Low ENergy Antiproton (ELENA). This will be capable, once fully operational, of slowing the electron from 1/10 of the speed of light to 1/500 of the speed of light. It is a synchrotron, a type of particle accelerator, and works similarly to a particle accelerator, but in reverse. Since the antiprotons have the opposite charge to protons, an accelerator which would speed up protons ends up slowing down antiprotons, allowing the physicists at CERN to manufacture antihydrogen atoms from these antiprotons. The majority of the slowing effect is, however, actually a result of the emission of synchrotron radiation, which is produced when a charged particle is accelerated in a curved path.
Antimatter is the densest fuel source in the universe. This is because, when antimatter comes into contact with matter, they annihilate, converting their mass into energy. he conversion ratio between mass and energy is expressed by the very famous equation E = mc^2. This equation states that 1kg of matter, if converted entirely into energy, yields 9*10^16J. Therefore, an antimatter bomb containing half a kilogram of antimatter would explode, under perfect conditions, with a yield equivalent to 1430 Hiroshima bombs. However, bombs are the least exciting use of antimatter. Antimatter's extremely high fuel density comes into use for very long-distance spaceship travel, where conserving fuel is very important. For it to be used as fuel, it needs to be contained.
Charged antiparticles can be effectively contained using a Penning trap. In a Penning trap, appropriately spaced magnetic fields exert a Lorentz force perpendicular to the direction of motion of the charged antiparticle and to the direction of the field. These fields both slow down the antiparticles by synchrotron radiation, and effectively prevent it from touching the sides and annihilating. Penning traps do not work for antihydrogen atoms. This is because antihydrogen atoms have a neutral charge overall, and so don't experience a resultant Lorentz force. As a result, a modified version of this, called a Penning-Malmberg trap is used.
The NASA Institute for Advanced Concepts is currently working on designing an antimatter rocket to send astronauts to Mars. For safety, they plan to use positron fuel instead of antiproton fuel. This is because positrons are much less massive than antiprotons, so the gamma rays produced as a result of annihilation will have much less energy, and therefore will be less harmful to the crew. Advantages of using antimatter fuel include higher fuel density, less moving parts, less parts needed for storage, and less danger to civilians if the rocket explodes.
The current NASA plan for a mission to Mars, the Mars Reference Mission, states that nuclear propulsion is the method of choice. Nuclear propulsion is preferred over chemical propulsion for this mission as it's much faster, so ironically the crew will be exposed to less radiation during the trip. If this rocket exploded, especially in the upper atmosphere, radioactive material would be spread over a large area. By contrast, if the antimatter rocket exploded, the gamma rays would dissipate almost immediately, and the greatest danger to bystanders would be the explosion radius, estimated to be around a kilometre from the spaceship. This is similar to the fireball created if a conventional rocket exploded.
Unfortunately, even the tens of milligrams of antimatter required for a Mars mission are very expensive. Positrons are probably the most cost-effective source of fuel for such a mission, at $250 million for 10mg. It must be taken into account that other missions will also cost large sums of money (each Saturn V launch cost $1.23bn), and antimatter rockets use far less materials for storage of fuel, so some costs may be reduced in this way. Therefore, an antimatter rocket is not a very far-fetched idea. Moreover, the cost of producing antiprotons is likely to decrease with further research, so the project may increase in viability over time.
Overall, building an antimatter rocket for a mission to Mars is an attractive idea, and may be used one day. However, the technology is so new as of now that the first mission will probably be made using more conventional technology.
Sources:
Commentaires